What aircraft owners should know about piston aircraft engine oil.
This is the first of a two-part article about the lubricating oil we use in our piston aircraft engines, and we’ll be covering a lot of territory. We’ll discuss the various types of engine oil—monograde versus multigrade, mineral oil versus synthetic—and the pros and cons of each. We’ll talk about aftermarket oil additives and whether they’re beneficial or just hype. We’ll touch upon oil consumption, optimum oil level, and how often to change the oil. We’ll also address oil filter inspection and spectrographic oil analysis, and how we use them to assess engine health.
Six Key Functions
If I asked you to explain the purpose of engine oil, I imagine you’d probably say something like “to lubricate moving parts and reduce friction and wear.” Now that’s certainly correct as far as it goes, but lubrication is only one of six key functions that oil must perform in your piston aircraft engine. In fact, the lubrication needs of our big displacement, slow-turning piston aircraft engines are really quite modest, compared for example to the high-revving engines in our automobiles. Lubrication demands tend to vary with the square of RPM, so a car engine has vastly more demanding lubrication requirements than an airplane’s engine does.
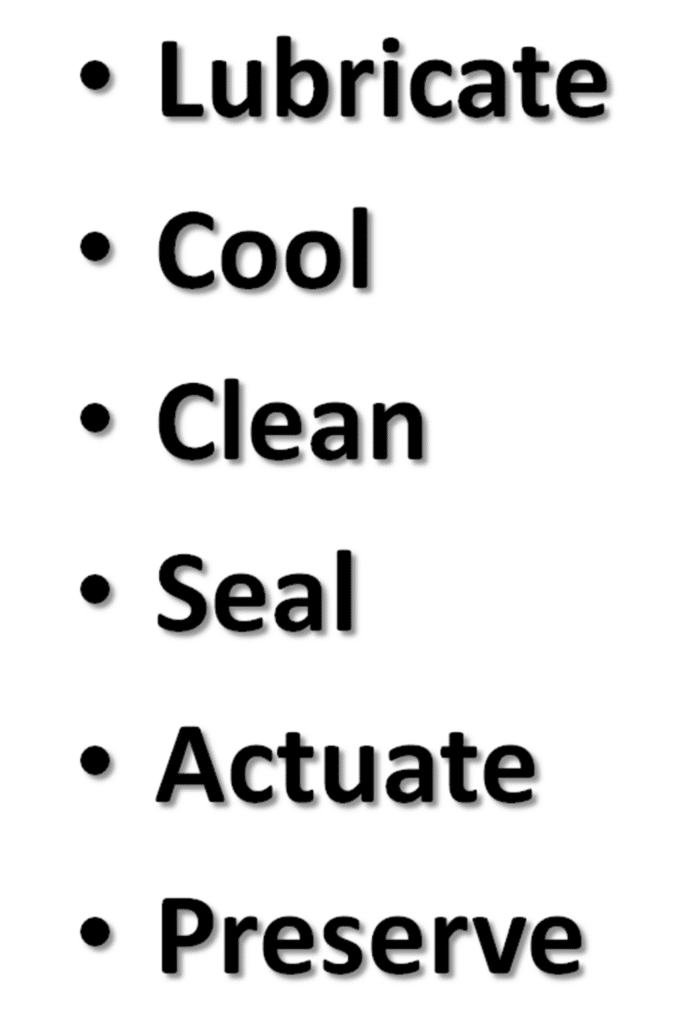
Oil also serves as a vital coolant for engine components that can’t be air-cooled. Pistons, for example, are exposed to just as much heat of combustion as cylinders, but they don’t have cooling fins or exposure to airflow. The only thing that keeps them from melting is the large quantity of oil that is splashed and squirted onto the bottom of the pistons to carry away the heat.
Another key function of oil is to keep the engine clean. Compared to car engines, piston aircraft engines are positively filthy creatures. They burn heavily leaded fuel and allow large quantities of lead salts, carbon, sulfur, water, raw fuel and other nasty combustion byproducts to blow by the rings and pollute the bottom end of the engine. The oil has to be able to keep these contaminants dispersed and hold them in suspension so that they don’t accumulate on internal engine parts in the form of sludge.
Oil also acts as a sealant to prevent the leakage of gases and liquids (including the oil itself) past piston rings, O-rings, gaskets, and various other kinds of seals.
If your airplane has a constant-speed propeller, oil serves as the hydraulic fluid that is used to adjust the blade pitch. If it is turbocharged, the wastegate is probably hydraulically actuated by oil as well.
Last, but certainly not least, oil is required to protect expensive components like crankshafts, camshafts, lifters and cylinder barrels from rusting during periods when the airplane is not being flown. Because we tend to fly our airplanes less often and more irregularly than we drive our cars, the preservative requirements of our aircraft engines are far more demanding than for automotive engines.
Friction, Wear, Lubrication
The purpose of lubrication is to reduce friction and wear on the engine’s moving parts. Friction occurs because even the smoothest surfaces have microscopic peaks and valleys. Whenever surfaces come in contact, these tiny peaks adhere to one another via tiny “micro-welds.” If those surfaces are in relative motion, the micro-welds constantly fracture and re-form, resulting in friction and wear. Friction is the resistance to relative motion and wear is the loss of material. Both are due to the fracture of the micro-welds.
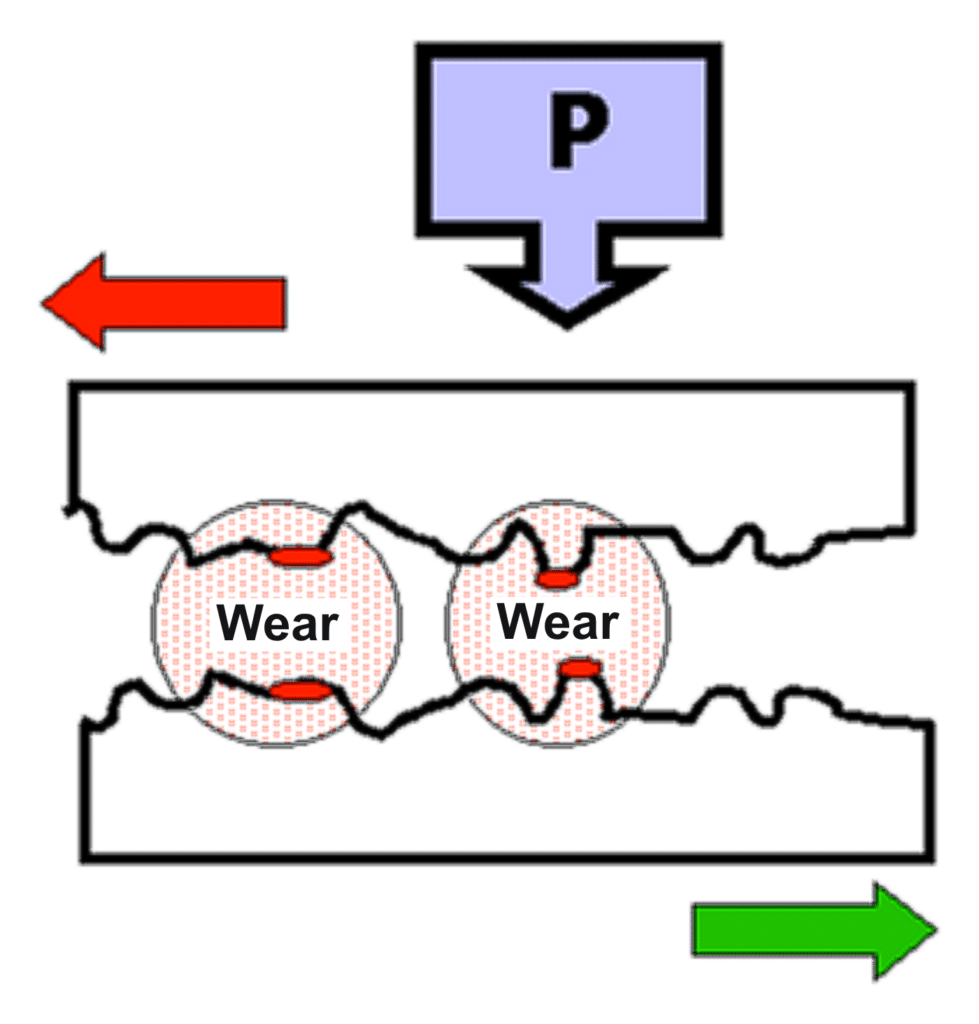
There are several different kinds of lubrication. The most effective kind is called “hydrodynamic” lubrication. It occurs when a fluid—most commonly a liquid like oil—is interposed between the moving parts. The relative motion of the parts creates sufficient pressure in the lubricant to keep the parts from touching.
Think of a water skier being supported by the skier’s relative motion to the lake and the resulting pressure of the interposing water. The water pressure prevents the skier from sinking and contacting the lake bottom. Or, consider a car hydroplaning on a rain-slicked road, its locked tires being separated from the pavement by water pressure. That’s hydrodynamic lubrication.
This kind of lubrication works well if the relative speed of the parts is high enough to overcome the load pushing them together. If the relative speed is not high enough, then there will not be enough lubricant pressure to keep the parts separated. (Think of the tow boat slowing down until the skier sinks.)
If hydrodynamic action cannot keep the parts separated, we must rely on another form of lubrication known as “boundary” lubrication. Boundary lubrication relies on a thin, soft, solid film deposited on the moving parts—typically by chemicals called “extreme pressure additives.” That thin film reduces friction and wear by chemically interfering with micro-weld formation.
Rub your hands together vigorously and you’ll feel the friction between your palms in the form of heat and resistance to movement. Believe it or not, you just created and then fractured a few zillion micro-welds on the surface of your palms! You can reduce the friction in several ways: for instance, by coating your palms with Vaseline, or by dusting them with talcum powder. Think of the Vaseline as hydrodynamic lubrication and the talc as boundary lubrication.
Pressure, Splash, Boundary
Most of the lubrication in our engines is hydrodynamic. It’s accomplished in two different ways. Some moving parts—such as the main and rod bearings—are “pressure lubricated” by oil that is distributed directly to those parts by means of a series of drilled passages in the crankcase and crankshaft known as “oil galleries.”
Many other moving parts—pistons, rings, cylinder barrels, cam lobes, lifter faces, gears—receive no direct lubrication from oil pressure. These critical components rely entirely on what is called “splash lubrication.” When the engine is running, lots of oil extrudes from the crankshaft’s pressure-lubricated main and rod bearings, and is flung in all directions by the rapidly turning crankshaft, splashing oil on everything in the vicinity.
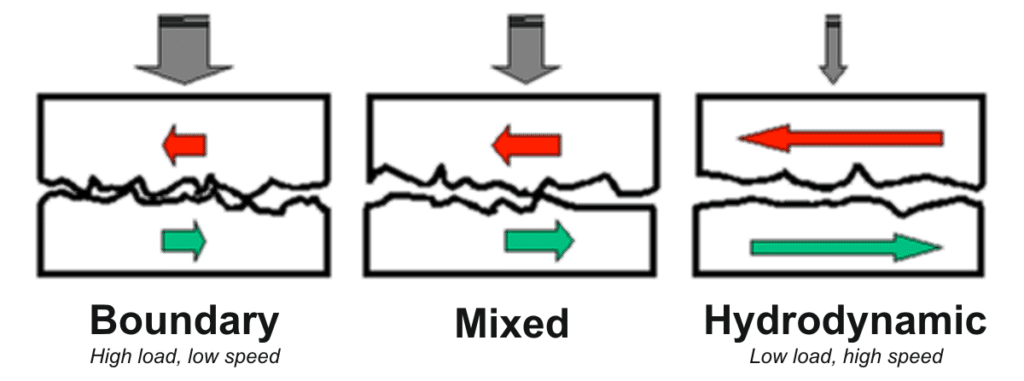
Other critical parts are too slow-moving and too heavily loaded to be separated hydrodynamically, and rely mostly on boundary lubrication. One interesting example is the interface between the piston rings and cylinder barrels. Most of the time, the piston is moving rapidly inside the cylinder, and the ring-to-barrel interface is lubricated hydrodynamically so there’s no metal-to-metal contact. At top-dead-center, however, the piston slows to a complete stop and then reverses direction. The slowing of the piston defeats any effective hydrodynamic lubrication in the critical ring-reversal area at the top of the stroke. For a brief but critical period during each crankshaft rotation, the rings stop moving, sink through the oil film—like a skier whose tow boat stopped—and come in contact with the cylinder walls. The only thing that protects this “ring reversal area” from accelerated wear is boundary lubrication.
Boundary lubrication is also crucial during the first few seconds after the engine is started. That’s especially true if the aircraft hasn’t flown for awhile and most of the oil has stripped off of the parts. Until oil pressure stabilizes and the crankcase fills with splash oil, dry engine parts have to rely on boundary lubrication alone.
Types of Engine Oil
Now let’s talk about the different types of oil, and the pros and cons of each. Here are the six most widely-used piston aircraft engine oils in the U.S.:
Multigrade oils: Aeroshell 15W-50 (50% synthetic) Exxon Elite 20W-50 (25% synthetic) Phillips X/C 20W-50 (0% synthetic) Monograde oils: Aeroshell 100/80 (non-AD break-in oil) Aeroshell W100/W80 (0% synthetic) Aeroshell W100 Plus/W80 Plus (0% synthetic) |
Aeroshell 15W-50 is by far the most popular multigrade oil. It dominates the GA market, although it’s my least-favorite oil (for reasons that I’ll address shortly). It is referred to as a “semi-synthetic” oil, because it is a 50-50 hybrid of petroleum-based mineral oil and a synthetic oil called polyalphaolefin or PAO. To this half-and-half mixture of mineral and synthetic base stock, Shell adds chemicals called “viscosity index improvers” to give the oil its multigrade properties. It also adds a complex additive package including ashless dispersants to help prevent sludge, corrosion inhibitors to help prevent rust, and an extreme-pressure boundary lubricant called butylated triphenyl phosphate (bTPP).
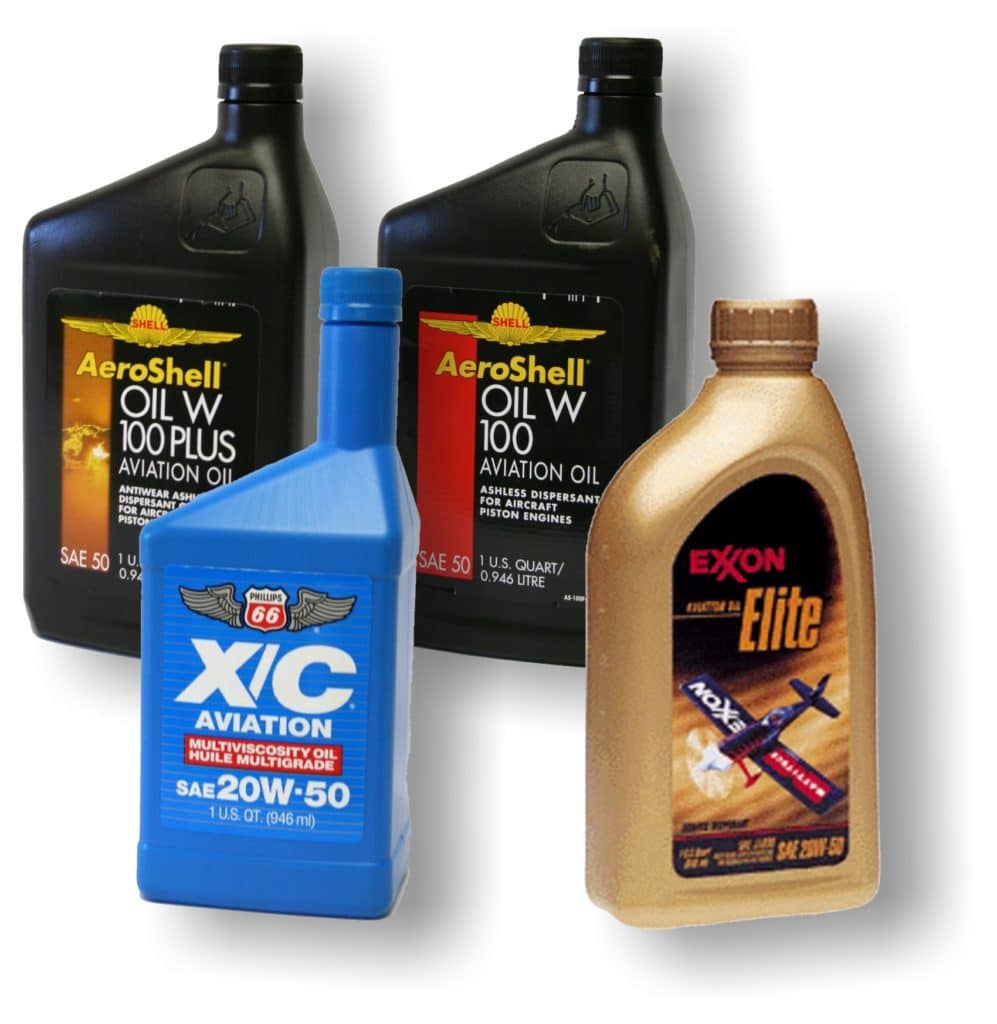
Exxon Elite 20W-50 is a very similar formulation to Aeroshell 15W-50, except that its base stock is only 25% synthetic and 75% petroleum-based. Its additive package is nearly identical to the one used in Aeroshell 15W-50.
Phillips X/C 20W-50 is an inexpensive, no-frills, all-petroleum multigrade. It’s made strictly from dead dinosaurs, with no synthetics, thank you very much. Phillips adds viscosity index improvers to obtain multigrade properties, and ashless dispersants to prevent sludge.
Moving on to the monograde oils, Aeroshell “straight 100” is an SAE 50 mineral oil with minimal additives. It’s used primarily as a break-in oil, and occasionally as an operating oil for radial engines.
Aeroshell W100 is by far the most popular monograde oil. The “100” means that its viscosity rating is SAE 50, and the “W” prefix means that it contains an ashless dispersant additive package to help keep particulates in suspension and minimize sludge formation. (This “W” prefix that denotes AD additives should not be confused with the “W” suffix in “15W-50” which denotes that SAE 15 is the oil’s “winter” rating.)
Aeroshell W80 is a less-viscous version of Aeroshell W100, and is used as a wintertime oil by operators who prefer monogrades.
Aeroshell W100 Plus is simply Aeroshell W100 to which Shell has added the same package of anticorrosion and antiscuff additives that they use in 15W-50.
Monograde vs. Multigrade
Monograde oil is simply mineral oil plus an additive package. It has viscosity, or thickness, that varies rather dramatically with temperature. At operating temperature—around 200 degrees Fahrenheit—it’s quite thin and flows very freely. But at room temperature, it’s thick and gooey. Get it cold enough and it won’t pour at all.
Multigrade oils are much less thick and gooey at cool temperatures. They still get thicker as temperature decreases, just not nearly as much. To make a multigrade oil, you start with a really thin monograde oil (something like SAE 10 or 15) and then add an artificial thickening agent called a “viscosity index improver” or VII. This is a man-made polymer that has the unusual property that it gets thicker and more viscous when heated—precisely the opposite of what mineral oil does. By combining oil with VIIs, the manufacturer can obtain pretty much any viscosity-to-temperature curve he likes. Multigrade aircraft oils are roughly 90% base stock, with the remaining 10% composed of VIIs and other additives.
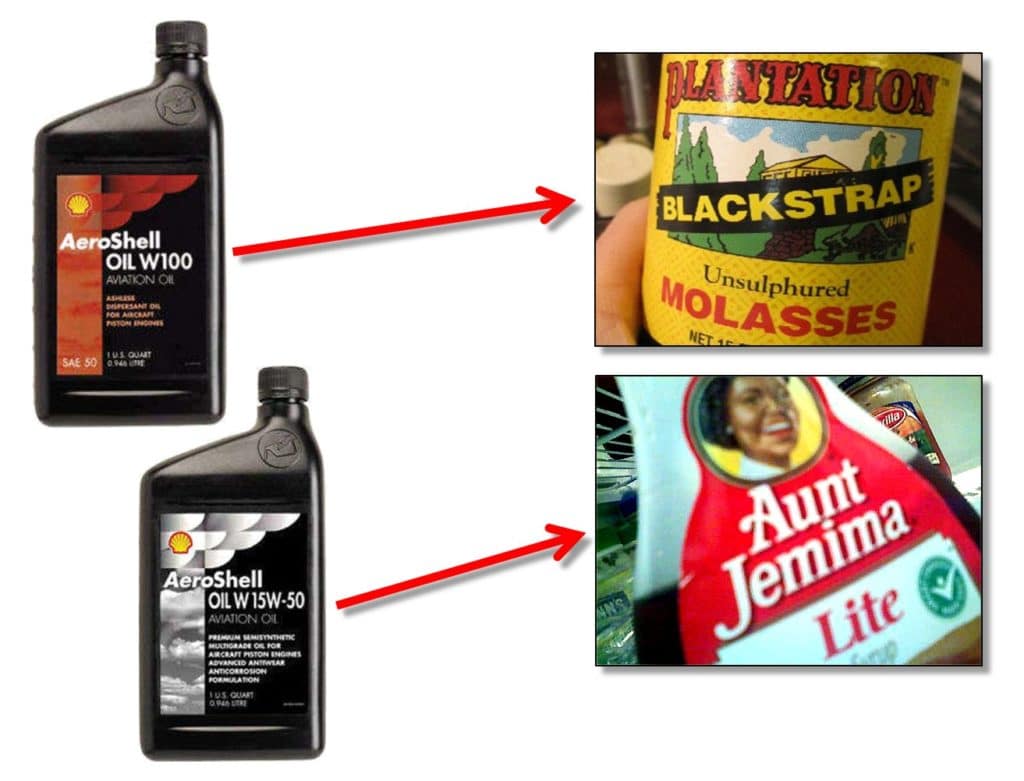
At operating temperature, Aeroshell W100 and 15W-50 have essentially the same viscosity. At room temperature or colder, the difference in viscosity is obvious and dramatic. The W100 pours like blackstrap molasses, while the 15W-50 pours like Aunt Jemima Lite. Or if you prefer, ketchup versus tomato juice.
Polymers
Oil is made up of giant molecules called polymers. Some are natural like mineral oil, others man-made like PAO. Different polymers have different shapes. The molecules of mineral oil have a lot of side branches, while the molecules of synthetic oil are smoother and less “branchy.”
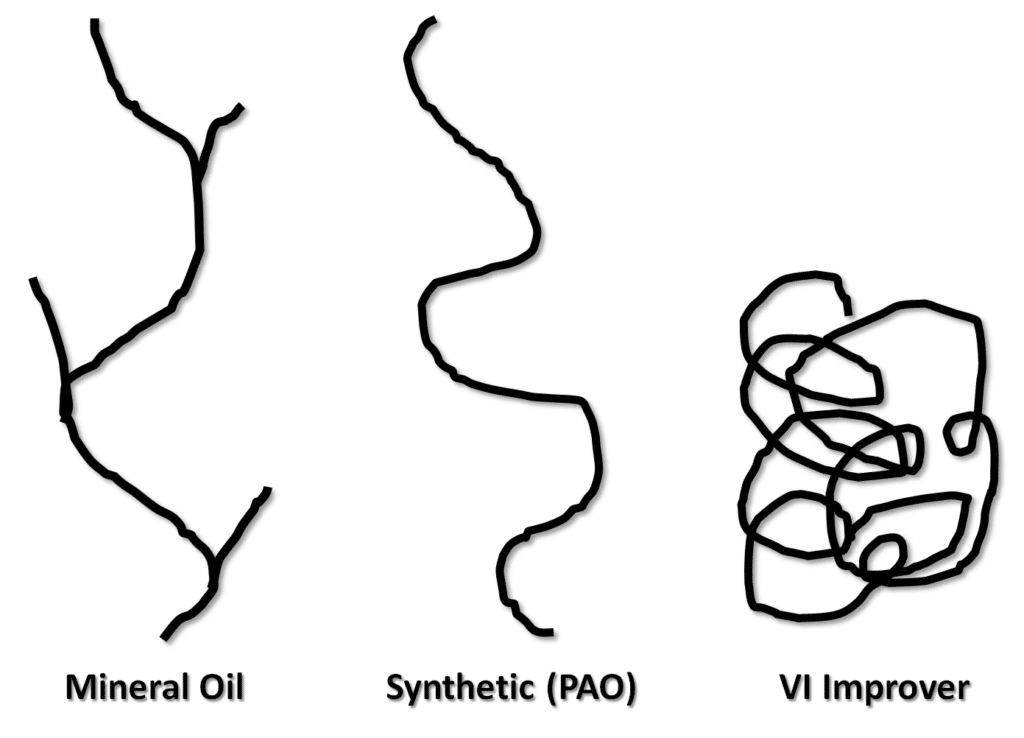
Mineral oil gradually degrades the longer it remains in service. The little branches gradually shear off the molecules—a phenomenon known as “polymer shearing”—and that causes viscosity to decrease. Because synthetic oil is less branchy, it suffers far less from polymer shearing and retains its viscosity longer. This means that synthetic oil can go a lot longer between oil changes, at least in automotive applications.
Viscosity index improvers are strange man-made molecules that roll up in a tiny ball when cool, but unroll and become more linear as temperature increases. That’s why thicken the oil at high temperatures.
Pros and Cons
The smooth, less branchy molecules of synthetic oils like PAO do offer some significant advantages over mineral oil. They provide improved lubricity, simply because they are smoother and more slippery. They also last longer, because they suffer less from polymer shearing and thermal breakdown.
But mineral oil also has its advantages. Its branchy molecules do a much better job of holding particulate contaminants in suspension so that they can be drained out at the next oil change instead of settling out as sludge. In fact, the full-synthetic Mobil AV 1 was withdrawn from the market more than a decade ago (in a hail of litigation from aircraft owners) because so many engines were ruined by lead sludge deposits. To put it bluntly, synthetic oil simply can’t deal with filth.
Mineral oil is also a better sealant, because its branchy molecules are less likely to sneak by O-rings and gaskets in the form of oil leaks.
The advantages of synthetic oils—which are quite compelling for automotive and turbine use—are much less significant for piston aircraft engines. The improved lubricity is less important simply because piston aircraft engines have such modest lubrication requirements. And the extended oil-change intervals that synthetics offer in automotive applications are of little use in piston aircraft engines, because the oil gets so filthy with blow-by that only an idiot would go more than 50 hours without draining the dirty oil.
Obviously, the advantage of multigrade oil is that it doesn’t thicken nearly as much at cold temperatures. This offers a significant benefit during cold weather if you have to start without a preheat. With multigrade oil, the oil flows more quickly to the main and rod bearings, and splashes more quickly onto the cam, lifters, pistons and cylinders.
On the other hand, the fact that multigrade oil remains thin and pourable at cool temperatures also can mean that it drains off engine parts more quickly after shutdown. You can see this for yourself by checking how much longer it takes for the oil level on the dipstick to stabilize after shutdown. It takes many, many hours with a thick monograde oil like Aeroshell W100, less with multigrade like 15W-50. Consequently, multigrade doesn’t provide as long-lasting a physical barrier against corrosive attack during extended periods of disuse. This is not important for “working airplanes” that fly every day or two, but can be important for airplanes that fly irregularly and sometimes sit unflown for weeks at a time.
Corrosion is the #1 reason that piston aircraft engines fail to make TBO. We almost never wear these engines out, we rust them out. This is a big problem in the owner-flown fleet, because owner-flown airplanes tend to fly irregularly. Working airplanes that fly every day or two almost always make TBO without breaking a sweat.
For these reasons, I personally prefer mineral oils over semi-synthetics for piston aircraft engines. The advantages of synthetics simply don’t benefit these engines the way they do automotive and turbine engines. I also prefer monogrades (like Aeroshell W100) over multigrades (like Aeroshell 15W-50), except when multigrade is necessitated by exposure to unpreheated cold-starts in sub-freezing temperatures. For aircraft operating is cold climates, I recommend using a mineral-based multigrade like Phillips X/C 20W-50 during the 4 coldest months of the year, and switching to a monograde like Aeroshell W100 for the remaining 8 months for maximum corrosion protection. Of course, if the airplane flies frequently and regularly so that corrosion risk is low, then there’s nothing wrong with using a multigrade all year around.
Aftermarket Additives
For as long as I can remember, there have been pitchmen promoting “miracle in a can” oil additives that claim to eliminate friction and wear, increase fuel economy, improve your landings, raise your IQ, and rescue your marriage.
The granddaddy of these is Marvel Mystery Oil. Folks have been pouring this red, sweet-smelling stuff into aircraft engines for more than 80 years. It was developed in 1923 by Burt Pierce, the inventor of the Marvel carburetor, and was intended as a fuel additive to clean carburetor jets. Why folks started using as an oil additive escapes me. The name “mystery oil” came from the fact that Burt Pierce refused to divulge its formula.
So far as I can tell, MMO doesn’t do any harm if used in moderation, but it doesn’t seem to do much good, either. Its formula is no longer a mystery since the Feds required the current manufacturer, Turtle Wax Inc., to publish the ingredients on a material safety data sheet. Turns out MMO has some interesting ingredients, including pig fat, perfume, and red food coloring in a base of petroleum distillate solvent. In case you were wondering, I don’t use MMO in my engines.
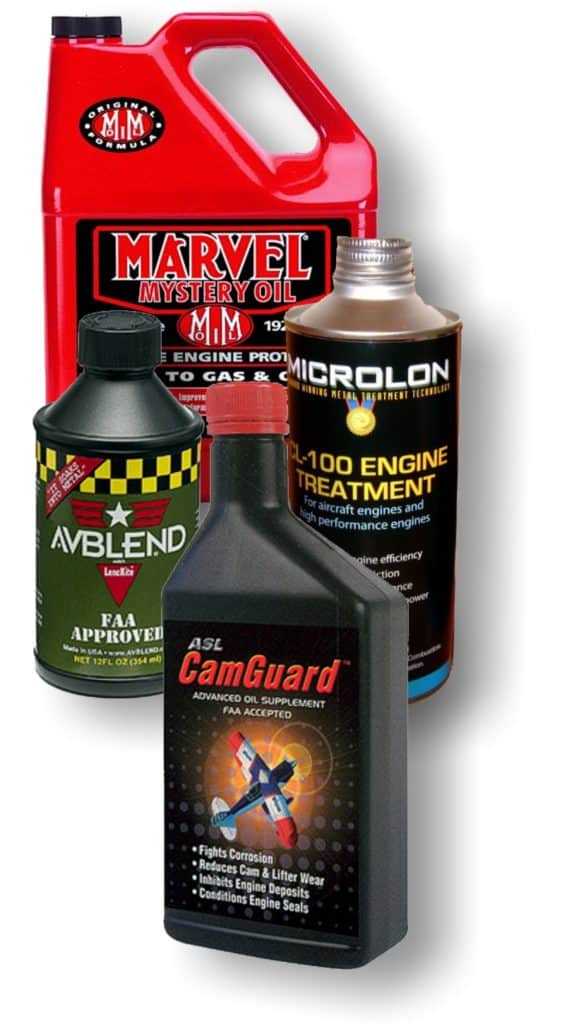
Some aftermarket oil additives—notably Microlon and Slick 50—contain a slippery resin called PTFE, which is made and marketed by DuPont under the tradename Teflon. The Microlon and Slick 50 folks claim that the stuff bonds with metal surfaces in your engine and virtually eliminates friction. This turns out to be pure bunk. Manufacturers of non-stick cookware will tell you just how difficult it is to get Teflon to bond to anything. Users of non-stick cookware will tell you just how easy it is to ruin the Teflon coating because it’s so fragile. NASA did a study of Teflon oil additives awhile back, and concluded that not only are they ineffective as a friction reducer, but they can actually damage an engine by clogging oil filters and tiny oil passages in hydraulic lifters. DuPont specifically warns against using Teflon in internal combustion engines. Nevertheless, the promoters of Microlon somehow convinced the FAA to approve its use in aircraft engines. My advice is to just say no.
AVBLEND has been around for two decades, and is a repackaged automobile oil additive called Lenkite that has been around for even longer. Back in the 90s, my friend Howard Fenton of Engine Oil Analysis in Tulsa ran a test of AVBLEND involving several piston twins that used AVBLEND in one engine and not in the other. When we looked at the oil analysis results for these airplanes, we could not tell which engine had the AVBLEND and which didn’t. Like MMO, AVBLEND appears to do no harm, but we can’t find any evidence of benefit either.
Given this dismal history of miracles-in-a-can, when another one called ASL CamGuard hit the market about five years ago, I was understandably skeptical. But in July 2007, I ran into CamGuard developer Ed Kollin at EAA AirVenture, got into a lengthy conversation with him, and discovered that he wasn’t your typical snake oil salesman. Turns out that Ed is a very sharp and experienced lubrication chemist who ran Exxon’s engine lab for many years, was heavily involved in the development of Exxon Elite, and seems to know more about aircraft oil and additives than anyone I’ve ever met.
After talking to Ed at length, I decided to run a test of CamGuard in my own airplane. I used it quietly for 18 months before telling anyone. Studying my oil analysis results, I found that the big spikes in iron (caused by rust) that I had always seen whenever the aircraft was idle for a month or more were completely eliminated. That got my attention. I also found a modest across the board reduction in other wear metals on the order of 10 to 20 percent. I examined the oil analysis history of several other airplanes using CamGuard, and saw much the same thing.
I’ve been using CamGuard in my engines for three years now, and I’m convinced the stuff works. We’re now using CamGuard in hundreds of airplanes managed by my company, all of which are on oil analysis, and we almost always see a clear reduction in iron and improvement in other wear metals once we start using CamGuard.
For most of the airplanes we manage, we recommend Aeroshell W100 with a pint of CamGuard added at each oil change. That’s what I use in my own airplane, and my two engines are at nearly 200% of TBO and still going strong. For wintertime operations in cold climates, I recommend Phillips X/C 20W-50 multigrade with a pint of CamGuard.
Oil Consumption
Take any group of aircraft owners, add a pitcher or two of beer, and it’s not long before they’re comparing notes on who’s airspeed is the highest and whose oil consumption is the lowest. The subject of airspeed is beyond the scope of this article. But I’m here to tell you that low oil consumption is highly overrated.
Lots of factors affect oil consumption. Six cylinder engines use more oil than four-cylinder engines. Big-displacement engines use more oil than smaller-displacement engines. TCM engines typically use more oil than Lycomings of similar horsepower. Chrome-plated cylinders use more oil than steel cylinders. Nickel-carbide cylinders use less oil than steel. Airplanes that fly a lot of short trips use more oil than ones that fly fewer long trips. And so forth.
Anything from a quart in 20 hours to a quart in 4 hours is in the normal range. I’ve seen engines that burned a quart in 4 hours throughout their entire life and made it past TBO without any problem. I’ve also seen engines that used hardly any oil and then wound up needing a top overhaul after 500 hours.
TCM doesn’t consider oil consumption to be a cause for concern until it exceeds a quart in three hours, and says that it isn’t an airworthiness issue until it exceeds about a quart per hour. Now, I don’t think any of us would allow our engines to get to the point of burning a quart per hour, simply because it’s so darned embarrassing to run out of oil before you run out of fuel.
I do get concerned about any sudden increase in oil consumption. If an engine has been using a quart in 12 hours for most of its life and suddenly starts using a quart in 6 hours, that means something has changed, and to me that’s a red flag. Until we figure out WHAT changed, we can’t be sure whether it’s serious or benign.
I also get concerned if the oil starts to turn dark and opaque quickly after an oil change—say, within 10 hours or so. That indicates excessive blow by, and we’ll want to do some testing and troubleshooting to determine why this is happening and which cylinder is the culprit.
Many owners install aftermarket air-oil separators to reduce oil consumption and keep the belly clean. I don’t like air-oil separators, because they return all sorts of ugly, nasty stuff to the crankcase that would otherwise be expelled out the breather. I think engine guru John Schwaner of Sacramento Sky Ranch says it best on his website: “Air/Oil separators are like hooking a line up to your anus and piping it back into your mouth. Excuse the crude analogy, but who wants the water, acids and other combustion residuals pumped back into one’s engine?”
Dipstick Level
Oil consumption can be exacerbated by filling the sump to the top mark on the dipstick. Many engines don’t like that, and will promptly toss a quart or two overboard until the oil level decreases to a level that the engine likes better. When an engine does that, it’s trying to tell you something.
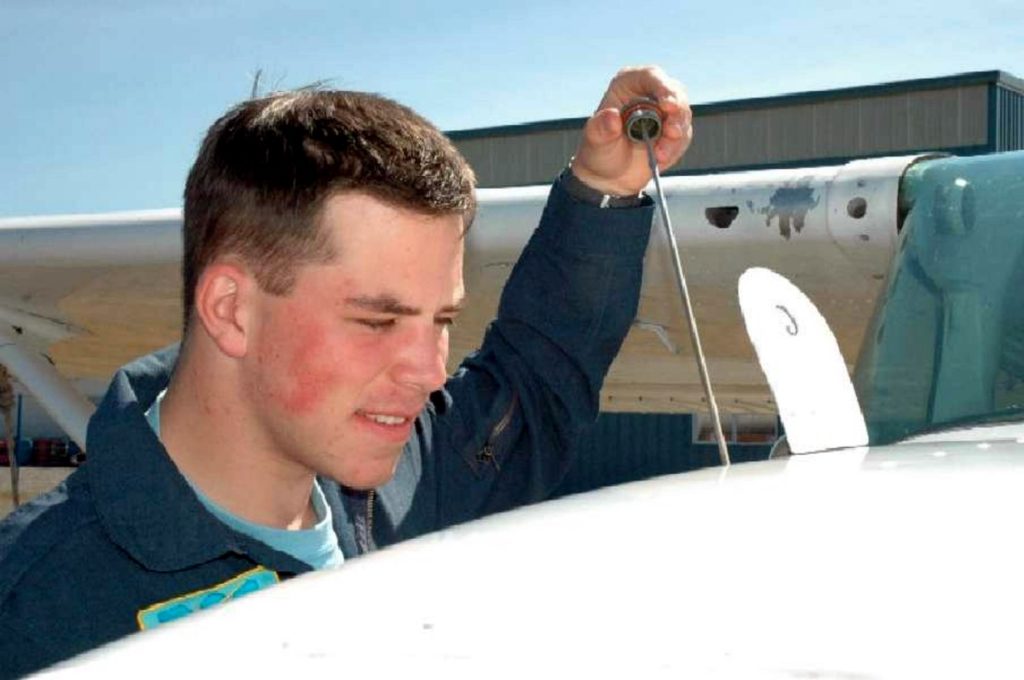
There’s no reason to fill the oil to the brim. Before an aircraft engine can be certified, the FAA requires the manufacturer to demonstrate that the engine runs just fine in all normal flight attitudes with the oil sump filled to just one-half capacity.
Actually, most engines can function with even less oil than that. If you’re curious how much less, just consult the engine’s type certificate data sheet, available on the FAA website.
For example, the TCM IO-550-N engine in a Cirrus SR22 has an 8-quart sump, but will run fine down to 3½ quarts. Most Lycoming O-320s also have an 8-quart sump but need only 2 quarts.
As a rule of thumb, we recommend operating most engines at about 2/3 of the maximum sump capacity. That means running 8-quart engines at about 5 or 6 quarts, and 12-quart engines at about 8 quarts. If your engine uses a lot of oil, you might want to add one more quart to those figures for a little extra cushion.
Oil-Change Interval
Because piston aircraft engines get the engine oil so filthy, regular oil and filter changes are an absolute must. Never go more than 50 hours or 4 months between changes, whichever comes first. (If you fly less than 150 hours a year, the 4 months will probably come first.) Some experts (like CamGuard’s Ed Kollin) recommend changing the oil every 25-30 hours or 3 months. If you have an older plane that has only an oil screen rather than a full-flow filter, you should not exceed 25 hours between oil changes.
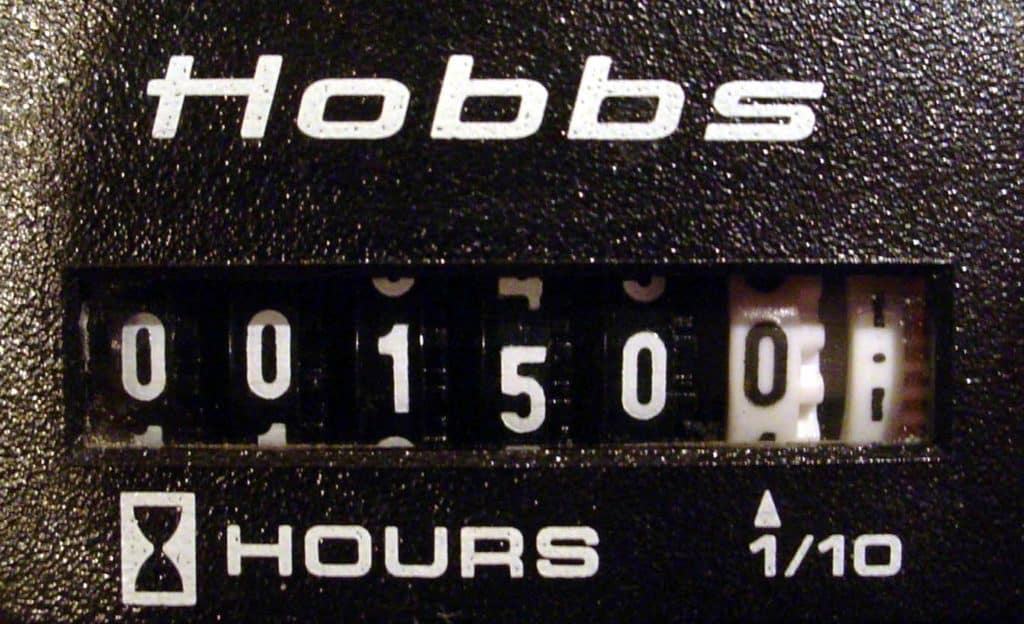
If appreciable metal is found in the oil filter, or if the oil analysis report comes back with any red flags, we will normally put the engine on a reduced oil-change interval until the source of the metal is identified or the problem resolves itself.
If you’re approaching an oil change and you know the airplane will go unflown for several weeks, it’s best to change the oil before the downtime rather than afterwards. Dirty oil tends to be corrosive, and you really don’t want your expensive crankshaft and camshaft bathed that stuff for any longer than necessary.
Oil Filter Inspection
Every time we change the oil, we also replace the oil filter, cut open the old filter, and carefully inspect the filter media for the presence of metal. Tossing an oil filter in the trash uncut is a capital offense.
We don’t get too excited about a few flecks of metal in the filter, particularly if it’s non-ferrous metal such as aluminum or copper or bronze.
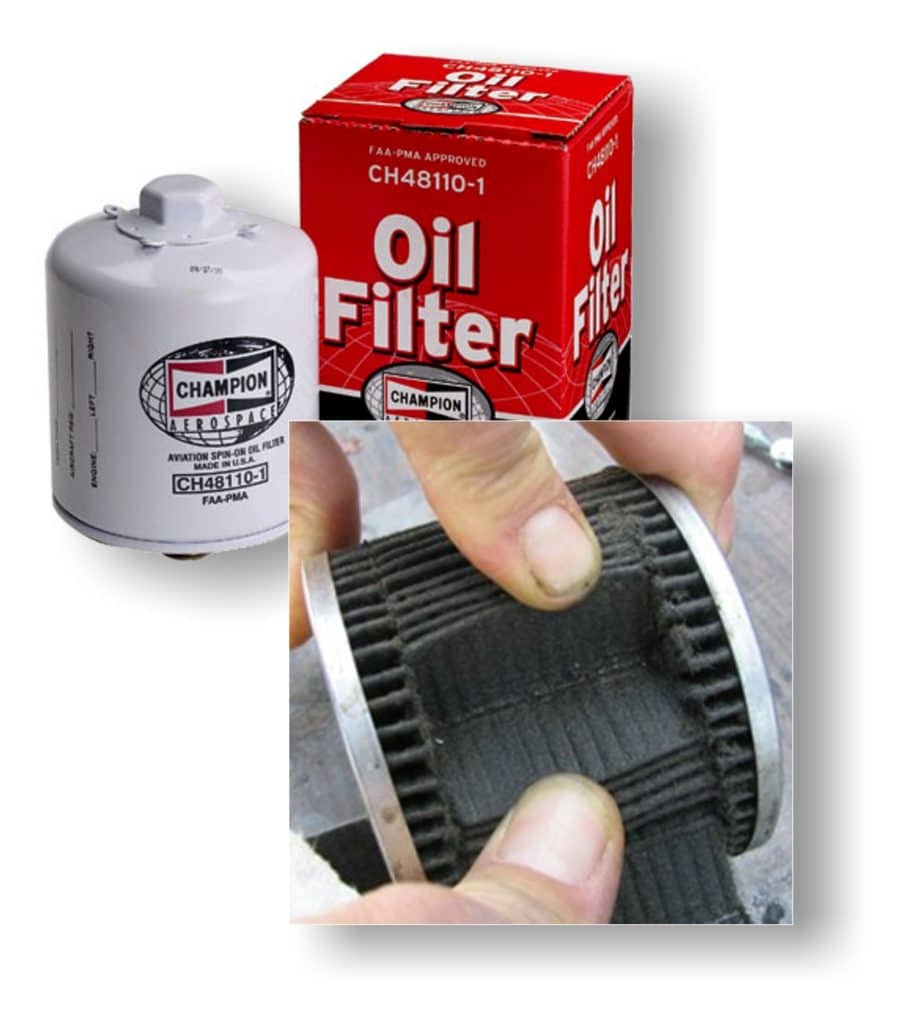
If there’s a significant amount of metal in the filter, we need to investigate to determine what kind of metal it is, and where in the engine it’s coming from. For example, metal whiskers (as opposed to flakes or particles) that are ferrous (attracted by a magnet) are typically associated with a failing lifter or cam lobe.
Of course, the source of the metal isn’t always so obvious. If we’re still not sure of where the metal is coming from after examining it with a magnifying glass and checking it with a magnet, the next step is to send it out to a lab for analysis under a scanning electron microscope. This isn’t nearly as scary as it sounds. It typically costs less than a hundred bucks and the results come back within a few days. (I recommend Aviation Laboratories in Louisiana for such analysis; www.avlab.com.) The SEM report will identify the exact quantity, shape, and type of metal, and usually the exact alloy. This is often sufficient to determine where in the engine the metal is coming from.
Oil Analysis
In addition to oil filter inspection, we also take an oil sample at every oil change and send it out for spectrographic oil analysis. This is not a substitute for filter inspection, but a complement. Inspecting the filter allows us to detect metal that is big enough to be caught by the filter and visible to the naked eye. Oil analysis allows us to detect microscopic metal particles that are small enough to pass through the filter and would be too small to see anyway. If we want to be confident that we will catch all abnormal wear events, we need to do both.
Oil analysis is superb at detecting very slow wear events that never throw off metal particles big enough to see. It’s also very good at providing early warning of accelerating wear events that would eventually show up in the filter or some other way, but not until significant damage is done.
I use and recommend Blackstone Laboratories in Ft. Wayne, Indiana (www.blackstone-labs.com) for oil analysis. There are lots of labs that do this work, but I think Blackstone is the best in the business. I’ve used Blackstone for years, and my company uses them for all of our managed-maintenance clients.
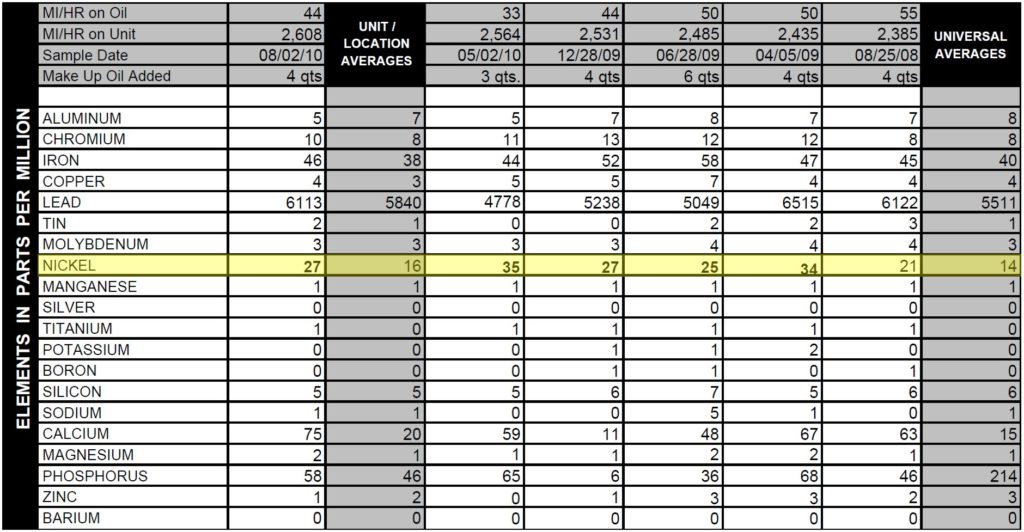
Here is an excerpt of an oil analysis report from Blackstone on the right engine of my Cessna 310. (I fly a twin, so of course I get two reports at each oil change.) The report for my left engine was unremarkable, but the one for the right engine was a bit more interesting.
The column on the left is the most recent sample, taken on August 2nd, 2010. The engine was then at 2,608 hours since major overhaul (more than 1,200 hours beyond TCM’s recommended TBO of 1,400 hours for this engine). Aluminum, chromium and iron all look great—those would be from pistons, rings, and cylinder barrels, respectively. Copper is fine as well—that would be from bearings or thrust washers. Lead is low, indicating that the engine does not have excessive blow-by. Silicon is also low, so the air filter is doing its job nicely. So far, so good.
The one red flag on this report is nickel, which is more than twice what it has been historically. The only source of nickel in my engines is exhaust valve guides, so there must be a valve guide that is experiencing accelerated wear. The elevated nickel has been present for at least two years, but is gradually increasing. Sooner or later, there will be a burned exhaust valve in my future and I’m going to have to pull a jug.
I did a compression test and borescoped all the cylinders, so I know that none of the exhaust valves are leaking or showing any abnormal heat signatures…yet. But the oil analysis is telling me that an exhaust valve problem is inevitable, so I’ll be watching closely. Of course, the oil analysis can’t tell me which cylinder is having the problem, but that’s something I’ll know in the fullness of time by means of continued borescope inspections and keeping a close eye on my digital engine monitor. Eventually, a distressed exhaust valve will become evident, and the corresponding cylinder will need to come off for repair.
You bought a plane to fly it, not stress over maintenance.
At Savvy Aviation, we believe you shouldn’t have to navigate the complexities of aircraft maintenance alone. And you definitely shouldn’t be surprised when your shop’s invoice arrives.
Savvy Aviation isn’t a maintenance shop – we empower you with the knowledge and expert consultation you need to be in control of your own maintenance events – so your shop takes directives (not gives them). Whatever your maintenance needs, Savvy has a perfect plan for you: