Can we prevent these catastrophic head-to-barrel separations?
On February 24, 2012, the National Transportation Safety Board issued Safety Recommendation A-12-7 to FAA acting administrator Michael Huerta, expressing great concern over 29 cylinder head fatigue failures of Titan-brand cylinders manufactured by Engine Components, Inc. between 2003 and 2009 and installed on Continental IO-520, TSIO-520 and IO-550 engines. The NTSB recommended that the FAA issue an Airworthiness Directive (AD) requiring repetitive inspections of these cylinders every 50 or 100 hours, and mandatory retirement of the cylinders at Continental’s published TBO.
If the FAA issues such an AD—and I hear rumors that the FAA is working on a Notice of Proposed Rulemaking (NPRM) as I write this article—it would affect more than 20,000 ECi Titan cylinders, and cost aircraft owners roughly $30,000,000.
It wouldn’t be the first such AD, and most likely not the last.
Déjà vu all over again
In May 2007—years before I became a columnist for EAA Sport Aviation—I wrote an article titled “Double Trouble” recounting the misadventures of a Cessna 182 owner who was flying at 6,000’ through the desolate Dakota Badlands when his 1,600-hour Continental O-470-U engine suffered a catastrophic head-to-barrel separation of the #1 cylinder. (See Fig. 1.) He was able to turn back and fly 20 nm on five cylinders to put the airplane on the ground at Dickenson, Mont. (KDIK) after losing about one-third of his engine oil.
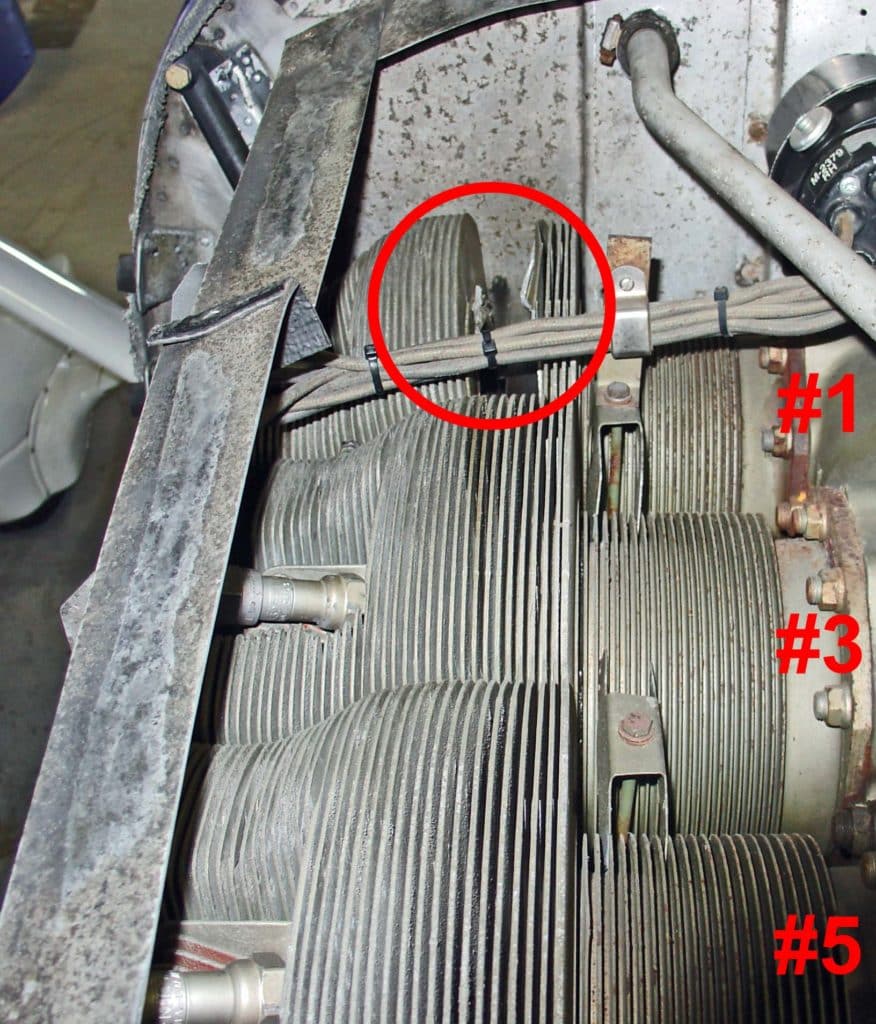
The mechanic at Dickenson Air Services had a replacement cylinder overnighted by FedEx and installed the next day by lunchtime. The Skylane owner did a 20-minute test flight with the new cylinder, followed by an inspection and leak check, and continued his trip…or so he thought. About 40 miles after takeoff, the same thing happened again! This time it was the #3 cylinder that blew its top. The owner made another emergency landing, this time at an unattended airstrip in Beach, N.D. This time, he’d had enough, and decided to replace the engine.
The cylinders that failed on this O-470-U engine were apparently Continental factory jugs. But cylinders from other manufacturers like ECi and Superior were also suffering similar fates from time to time. In fact, it wasn’t long afterwards that the FAA had Superior Millennium cylinders boresighted in their rulemaking machinery.
The Superior Millennium AD
On April 11, 2008, the FAA issued an NPRM announcing their intention to issue an AD against all investment-cast Millennium-brand cylinders manufactured by Superior Air Parts and installed on Continental IO-520, TSIO-520 and IO-550 engines. The proposed AD would require repetitive 50-hour compression and soap-bubble testing of all investment-cast Millennium cylinders with more than 750 hours in service, and mandatory retirement of such cylinders at Continental’s published engine TBO. The cost of compliance to aircraft owners was estimated by the FAA at $12,400,000.
Many of us in the industry (including me) were outraged by this NPRM. First, there had only been 24 reported failures of these Superior Millennium cylinders out of a population of some 8,000 cylinders, giving a failure rate of 0.3%—lower than the historical failure rate of Continental factory cylinders. Second, most of the reported failures occurred in Alaska-based airplanes operated by a single commercial operator in operations involving extraordinarily high numbers of maximum-performance takeoffs and landings per hour. Finally, these investment-cast Superior Millennium cylinders were—in the judgment of many piston aircraft engine experts—the best built, most durable, most efficient cylinders that had ever been offered for big-bore Continental engines. The FAA was proposing to legislate all of them out of existence because of a relatively trivial number of failures experienced almost exclusively by one operator in Alaska. Good grief!
Predictably, a hue and cry arose from the industry. Hundreds of comments were submitted to the FAA’s rulemaking docket, almost all of them opposing adoption of the draconian proposed AD. Nevertheless, the FAA would not be deterred. On August 5, 2009, it issued the Final Rule on AD 2009-16-03 tolling the death knell for Superior investment-cast cylinders, and penalizing thousands of aircraft owners whose only crime was deciding to spend a bit more money to install top-of-the-line cylinders on their engines. If you ask me, this AD was a real travesty.
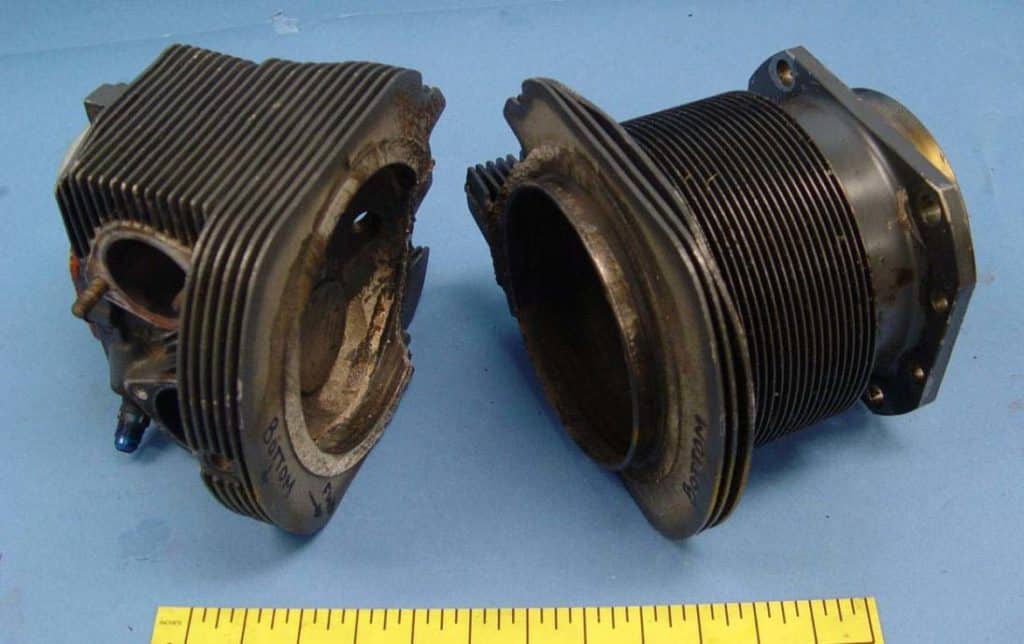
Now they’re after ECi Titans
Fast forward to the present: NTSB Safety Recommendation A-12-7 and the FAA NPRM that will likely result would probably have the same impact on owners of 20,000 ECi Titan cylinders as AD 2009-16-03 had on 8,000 Superior Millenniums. The cost to owners would be staggering, roughly $30 million by my reckoning. And to what end?
According to figures furnished to the FAA by ECi, the lion’s share of the affected cylinders would fall into three groups. One group of 7,797 cylinders manufactured in 2003 and 2004 installed on Continental IO-520 and TSIO-520 engines had 15 reported failures for a failure rate of 0.19%. Another group of 12,339 cylinders manufactured from 2005 to 2008 installed on TSIO-520 engines has had 9 reported failures for a failure rate of 0.07%. A third group of 5,232 cylinders manufactured from 2005 to 2008 installed on IO-520 engines has had no reported failures and a perfect record to date. These failure rates are extraordinarily low—lower than those seen in investment-cast Superior Millenniums, and lower than the historical norms for Continental OEM cylinders.
By any historical measure, these ECi Titans have proven themselves to be extremely reliable—but apparently not reliable enough for the NTSB and FAA. I can’t help but wonder just how low a failure rate it would take to satisfy the Feds. Is it technically possible to build a totally failure-proof cylinder? Even if it is, could we afford to buy them? Do you suppose that the $30 million that an AD against ECi Titans would probably cost aircraft owners might be better spent on something else…perhaps recurrent training to make those owners safer, more proficient pilots?
Don’t mind me, I’m just thinking aloud.
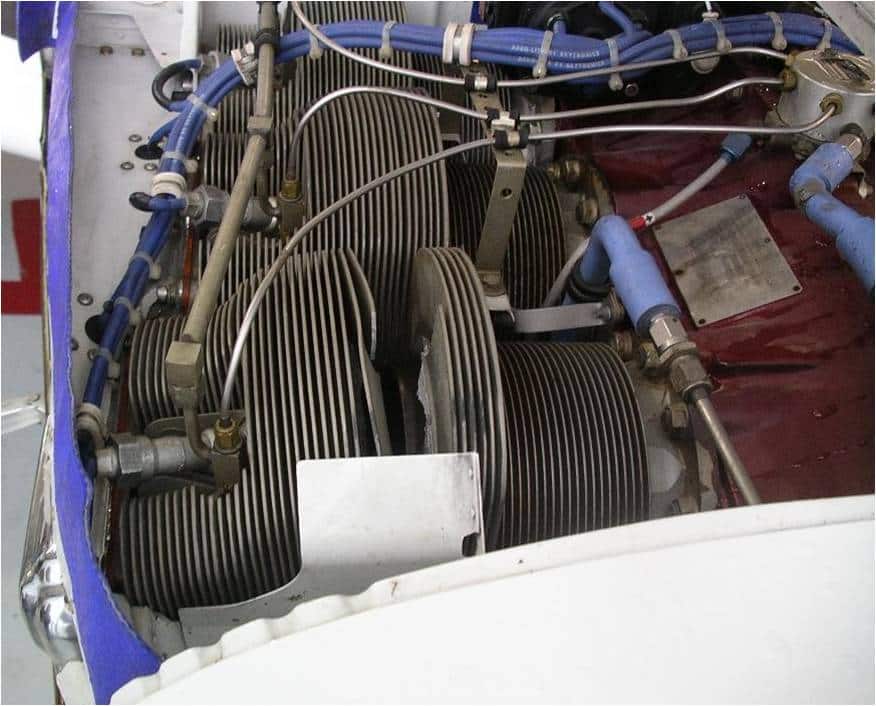
Why do they fail?
Rare as these head-to-barrel separation failures are, it’s important to understand what makes them happen. Recently, I’ve had the opportunity to discuss the matter at considerable length with ECi’s legendary chief engineer Jimmy Tubbs, and to study the results of research studies performed by his engineering group in San Antonio. As a result, I’ve become convinced that the key to preventing these failures doesn’t lie in more FAA rulemaking or in building more robust cylinders, but rather in educating the pilots and aircraft owners who fly behind these cylinders.
Any aircraft cylinder—whether made by Continental, Lycoming, Superior or ECi—will fail eventually if allowed to remain in service long enough. Cylinder heads are made of aluminum alloy, and like all non-ferrous metals they have a finite fatigue life. A fundamental principle of Metallurgy 101 is that any non-ferrous metal will ultimately fail from fatigue if subject to enough repetitive stress cycles. (This is not true of iron, steel and titanium, which theoretically can survive forever without cracking if subject to repetitive stress that does not exceed a threshold called the “fatigue limit.”) Fatigue failure of an aluminum alloy cylinder head is not a matter of “if,” only a matter of “when.”
We know these cylinders can last for a long, long time. Case in point: 9 of the 12 cylinders on my 1979 Cessna T310R have nearly 4,800 hours total time in service, which is 3.4 times the 1,400-hour TBO that Continental publishes for my TSIO-520-BB engines. They have been in continuous service for 33 years. Why have my cylinders lasted so long while others have failed catastrophically before attaining even one TBO?
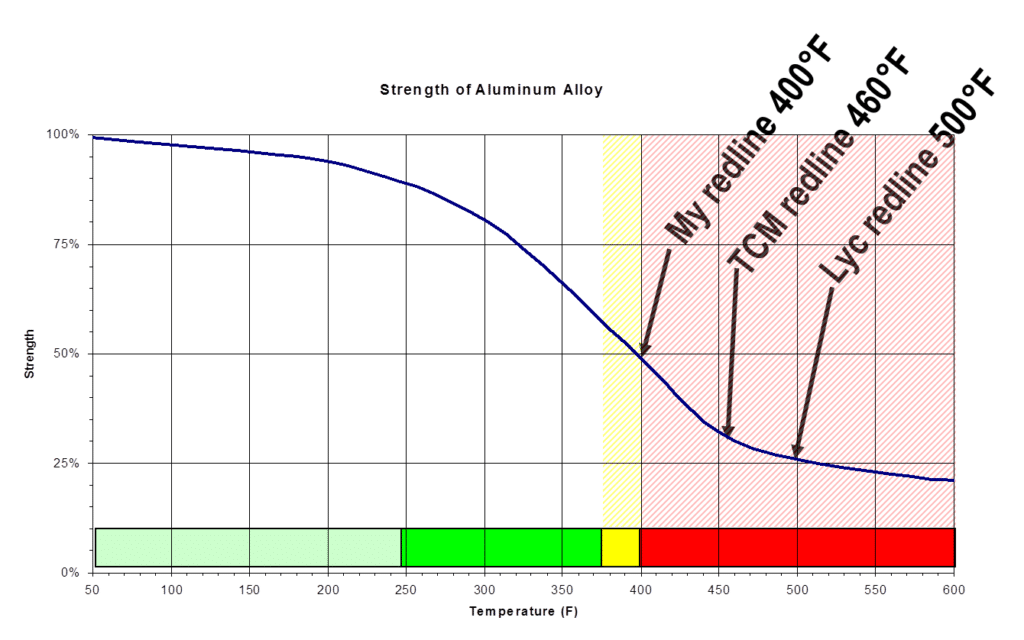
I’ve long been convinced that the answer to cylinder head longevity lies in keeping the heads cool. The strength of aluminum alloys drops sharply as temperature increases. (See Fig. 4.) At 400°F, the head casting loses half of its strength; by 500°F, it loses three-quarters of its strength. That’s why I’m obsessive about keeping my CHTs well-controlled. I always try to keep all CHTs at 380°F or less. The CHT alarm on my JPI engine monitor is set to go off at 390°F, and when it does, I take immediate action to bring the CHT down: I lower the nose if I’m climbing, open the cowl flaps, richen the mixture if I’m ROP, lean more if I’m LOP…whatever it takes.
But ECi’s research suggests that this loss of tensile strength at elevated CHT is only part of the story. To understand the rest of the story, we need to know something about the anatomy of the junction that attaches the cylinder’s aluminum head casting to its steel barrel.
Anatomy of the junction
The head-to-barrel junction is a threaded interface, with internal threads machined into the head mated to external threads machined onto the barrel. During manufacture, the head casting is heated to about 650°F, while the barrel is chilled. The hot head and the cool barrel are then quickly screwed together until the top of the barrel bottoms out against the abutment surface of the head casting. As the temperatures of the head and barrel equalize, the head shrinks and the barrel expands to create an “interference fit” that locks the two together permanently. (See Fig. 5.)
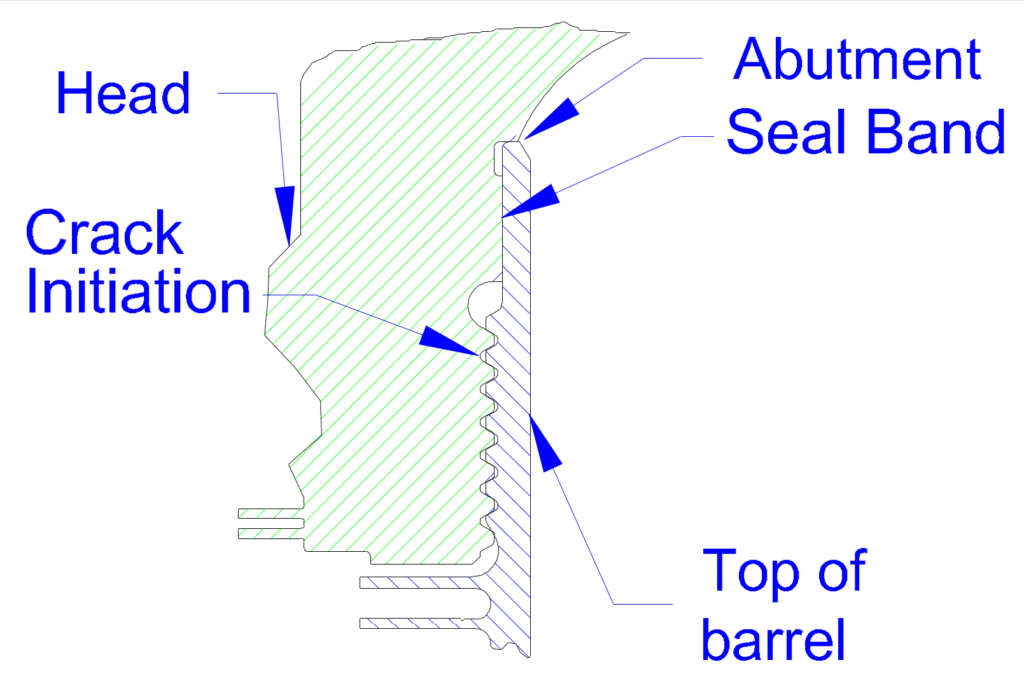
I’d been under the mistaken impression that it was the threads that held the head and the barrel together, but it turns out that’s wrong. The real strength of the head-to-barrel junction lies in the non-threaded area above the threads, variously known as the “seal band” or “friction band” or “shrink band.” It is primarily the friction of the interference fit in the seal band area that gives the junction its strength. In fact, ECi’s current specs provide for a slightly tighter interference fit at the seal band than at the threads (by a few thousandths of an inch), because their testing revealed that this creates a more robust junction.
The “tightness” of the interference fit is a function not only of manufacturing tolerances, but also of temperature. As the cylinder temperature rises, both the head and barrel expand. But because the coefficient of expansion of aluminum is about twice that of steel, the head expands twice as much as the barrel. Thus, as CHT increases, the interference fit of the head-to-barrel junction loosens. If the CHT becomes high enough, the seal band can start to lose its grip, transferring stress to the threaded portion of the junction. When that happens, the top thread takes the lion’s share of the stress, and ultimately a crack will initiate at that location that can grow over time to the point that the junction fails catastrophically.
How hot does the CHT have to be before this happens? There are two major factors that affect the junction’s tolerance for high CHT. We’ve already mentioned one of them: The amount of interference fit machined into the parts during manufacture. A second factor identified by ECi’s studies—and a surprising one—is that junction strength is adversely affected by high blow-by past the compression rings, particularly if the new cylinder is not properly broken in and the barrel becomes glazed before break-in is complete. High blow-by appears to weaken the head-to-barrel junction because the associated hot gases heat up the junction and impair the interference fit without being reflected on the CHT gauge (which measures cylinder head temperature at a location quite distant from the junction). Thus, a high-blow-by cylinder will have a hotter junction temperature (and thus a weaker junction) than a low-blow-by cylinder with the same indicated CHT.
Preventing these failures
After reviewing all the data, I can’t help but conclude that these head-to-barrel separation failures would be drastically reduced—perhaps even eliminated altogether—if we could persuade pilots to keep their CHTs under control, and teach them how to break in new cylinders properly. A big part of this is pilot education: So many pilots see that the green arc on their CHT gauge extends all the way up to the red-line at 460°F for Continental engines and 500°F for Lycomings, and think that there’s nothing wrong with running their CHTs well up into the 400s. Wrong! We need to teach them the critical importance of proper temperature control.
Another huge part of the problem is lack of proper CHT instrumentation. There are still way too many airplanes flying—roughly half of the piston-powered fleet—with nothing but the original factory CHT gauge that instruments only one cylinder and leaves the remaining 3 or 5 cylinders unmonitored. To make things worse, this legacy CHT instrumentation is seldom calibrated and often wildly inaccurate, sometimes displaying CHT values as much as 50°F less than what it actually is.
I’m convinced that if every piston-powered aircraft was equipped with a modern probe-per-cylinder digital engine monitor that set off an alarm anytime any CHT rose above 390°F, these head-to-barrel separation failures would become a thing of the past.
You bought a plane to fly it, not stress over maintenance.
At Savvy Aviation, we believe you shouldn’t have to navigate the complexities of aircraft maintenance alone. And you definitely shouldn’t be surprised when your shop’s invoice arrives.
Savvy Aviation isn’t a maintenance shop – we empower you with the knowledge and expert consultation you need to be in control of your own maintenance events – so your shop takes directives (not gives them). Whatever your maintenance needs, Savvy has a perfect plan for you: